Plate Tectonics, Subducting Slabs, and Nature Papers
I've mentioned before in this blog that the Earth is made up of continental and oceanic plates. At convergent plate margins, less buoyant oceanic plates are subducted beneath the continental plates, and drawn down into the mantle. Although the mantle is often depicted as convecting molten lava, it is actually solid rock. The reason that the solid rock in the mantle is able to convect however, is due to the immense stress that it is subjected to over huge periods of geological time.
The way a slab behaves once it begins its descent into the mantle is poorly understood. The only evidence we have of the shape of these subducted slabs at depth is from seismic imaging1, where the colder, more rigid slab is visible amid the hot, convecting mantle. These images, however, are often difficult to interpret. Moreover, they only offer us a snapshot in time, while tectonic processes span unimaginably long geologic timescales. For this reason scientists often disagree on the angle of trajectory for the descending oceanic plate.
A Case Study
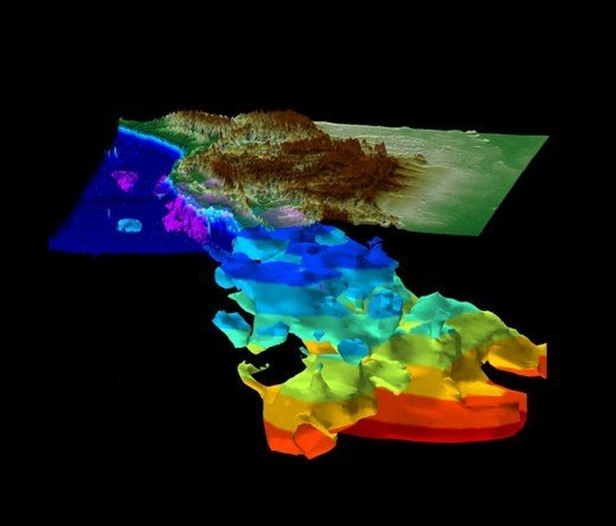
Image 1: Slab wall imaged underneath North America (Credit: Sigloch; http://www.geophysik.uni-muenchen.de/Members/sigloch (see also ref. 2)).
As North America moved westward after the initial breakup of Pangea2 ~200 million years ago, the Panthalassa Ocean to its west began to close. For many years, geologists believed that the Farallon plate that lay beneath the Panthalassa Ocean, was subducted eastwards into a trench at the western edge of North America. In an exciting new paper, however, geologists Dr Karin Sigloch and Dr Mitchell Mihalynuk have proposed a new theory for the history of the slab that lies beneath North America. After analysing high resolution topographic images of the slab (see image 1), they found that its horizontal cross-sectional shape did not match the contours of the western coast of North America. Moreover, the slab is a massive 400-600km across, 4-6 times thicker than would be expected from a single sheet of subducting lithosphere3 . The plate appears to have been subducted at a close to vertical angle, and so there became a need to explain its unexpected thickness. The vertical appearance of the subducted plate further contradicted a westward-moving trench along the west coast of North America. There also appears to be a second phase of subduction, a younger (shallower) section of slab wall which lays further west, and connects up to present day subduction under North America.
One way in which the geologists were able to explain these phenomena was by the presence of a volcanic arc system within the Panthalassa Ocean (the ocean that existed to the west of North America at this time). If there had been a string of island arcs, the Farallon slab could have subducted to the west, into a stationary trench along the archipelago (see image 2). This would explain the discontinuity between the shape of the subducted slab wall and the shape of the west coast of North America. Once the Panthalassa Ocean that lay to the east of the archipelago-associated trench had closed, North America would have collided with the island arcs, and a 'polarity switch' would have occurred. Now, the remaining ocean that lay to the west of the arcs would be subducted to the east, underneath North America.
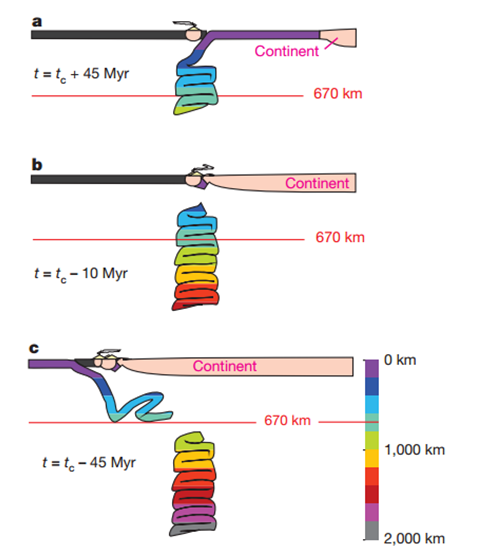
Image 2: (a) Westward subduction and slab pile-up under volcanic arc, (b) collision of North America with the volcanic arc, and closure of the ocean that lay east of the arc system, (c) polarity-shift in subduction, and subduction of the remaining ocean in an easterly direction (Credit: Reference 1).
An explanation for the huge width of the slab was also proposed in this paper, and has far-reaching implications for future studies of slab dynamics. As already discussed above, the mantle flows. At approximately 670 km, there is a viscosity4 jump within the mantle, where the mantle below is much more viscous than above. The theory here is that the subducting slab moves relatively easily through the less viscous upper mantle, but begins to buckle and fold onto itself as it reaches this boundary. There may even be a period of time where the slab is not dense enough to continue its descent into the lower mantle, and would therefore have to 'wait' at the boundary until there is enough material to allow the slab to pass through into this higher viscosity environment.
Please follow this link *doi:10.1038/nature12019* to read more about this exciting new theory!
1Seismic imaging distinguishes between the cold rigid slab and the hot convecting mantle by the speed at which the P-waves travel through it. P-waves are able to travel faster through colder, denser material.
2Pangea was a supercontinent that formed ~300 million years ago during the Late Palaeozoic, and existed for just over 100 million years, before it began to break up during the Mesozoic.
3Lithosphere comprises the Earth's crust, and some of the uppermost mantle.
4Viscosity is the extent to which a substance resists uniform flow. For example, honey is more viscous than water.
Bibliography
- Sigloch, K., & Mihalynuk, M.G., Intra-oceanic subduction shaped the assembly of Cordilleran North America. Nature. 496. 50-57 (2013) (doi:10.1038/nature12019).
- Sigloch, K., McQuarrie, N., & Nolet, G. Two-stage subduction history under North America inferred from multiple-frequency tomography, Nature Geoscience, 1, 458 – 462 (2008) (doi:10.1038/ngeo231).
--
__._,_.___
No comments:
Post a Comment